Fig. 1
General 19F MRI cell labeling . (a) A general protocol for 19F MRI of labeled cells involves ex vivo labeling of the cells, transfer to the subject, and then imaging. A smaller number of studies use direct in vivo labeling, typically macrophages. (b) “Hot-spot” imaging. The figure demonstrates the images typically obtained with T1, T2 contrast agents, and a 19F label. The contrast agents change the contrast in the anatomic (1H) scan, while the 19F image must be acquired separately, rendered in false color, and is then typically superimposed over the standard anatomic scan (in gray scale). Thus, the detection of 19F signal is less ambiguous, and, more importantly, the signal intensity directly relates to agent concentration, given sufficient signal to noise. In practice, this means that the cell numbers can be estimated directly from image data, one of the main advantages of the technique
In the last decade, “hot-spot” imaging has gained a lot of attention. Hot-spot imaging is hetero-nuclear MRI using 13C, 23Na, 31P, or 19F in addition to 1H, which enables direct detection rather than indirect, as is the case for CAs (Fig. 1b). Out of these four elements, 19F has been the main focus as it is essentially absent in biological tissues; thus there is no endogenous background signal, which makes it more attractive for hot-spot imaging [2]. One clear example of the advantage of the lack of background signal is a recent paper using 19F MRI to track inflammatory macrophages and monocytes in a murine model of multiple sclerosis [3]. Here the inflammatory cells were found not only in the diseased spinal cord, as expected, but also in neighboring bone marrow tissue. This ability to locate 19F signal even in unexpected locations without comparison to a “before” image, given a sufficient signal-to-noise ratio, is one of the key advantages of the technique.
19F MRI has been applied as a powerful imaging tool for diverse applications , such as cell tracking [4], drug metabolism, plaque detection, and monitoring inflammation. Cell tracking using 19F MRI has been applied to various cell types, such as dendritic cells (DCs), T cells, stem cells, and macrophages [5]. In order to distinguish relevant cells from the background, they need to be labeled with fluorinated labels, described in the following section.
2 Fluorocarbons
2.1 General Properties of Fluorine
Using fluorine in MRI has several advantages as 19F is a spin ½ nucleus, which means that it has an odd number of protons and neutrons and spherical charge distribution, and a gyromagnetic ratio close to the proton. Thus, for a given magnetic field value, the spin processes at almost the same frequency, permitting the use of a conventional proton spectrometer to detect fluorine [6]. Fluorine has 100 % natural abundance and an extremely broad chemical shift and is not naturally present in biological tissues and therefore has no biological background signal; the traces of fluorine in bone and teeth exhibit a very short spin-spin relaxation time (T2) and are not visible to conventional MRI methods [2]. In all, fluorine MRI gives a very high contrast-to-noise ratio and specificity, in a situation where a fluorinated element is introduced as a contrast agent . Such agent requires a very high density of 19F nuclei on the molecule in addition to a high tissue concentration in order to produce an image quality such as the one of 1H MRI. However, it is important to realize that the administered levels of 19F will still be many orders of magnitude lower than the amount of 1H present in biological tissues, and thus, despite all its advantages in terms of imaging properties, the low concentrations of 19F frequently result in images that hover at the detection limit with low signal-to-noise ratios (SNRs). Finally, some fluorocarbons show a T1 sensitivity to oxygen tension, which may allow its use as an in vivo sensor [2]; alternatively, this T1 variability can be a nuisance, hindering accurate quantification.
2.2 Fluorinated Compounds/Molecules
An example of fluorinated compounds includes fluorinated peptides, polymers, and also fluorinated small molecules, such as sugars; however these result in much lower cell loading and, as a result, a lower detection sensitivity. Small fluorinated molecules have lower 19F content than larger fluorocarbons, such as perfluorocarbons (PFCs); however their advantage is that they can be used to study cell functionality [5]. Despite this benefit, the insufficient sensitivity of the small molecules makes them less attractive for in vivo use. Recent developments in small fluorinated molecules include fluorinated albumin [7] and self-assembling nanoparticles sensitive to their environment [8], although neither of these have been applied to cell tracking . Some examples are listed in Table 1.
Table 1
Overview of different 19F labels, cell types, and cell loading
Major 19F component | Additives | Hydrodynamic diameter (nm) | Cell type | Average cell loading (×1012 19F atoms/cell) | No. of (dominant) resonances | Ref. |
---|---|---|---|---|---|---|
Various PFCs | Atto 647 | 200–300 | Primary human DCs | 30 | 1 | [22] |
PFCE PFOB | 233 224 | Primary human stem/progenitor cells | 20 0.5 | 1 Multiple | [33] | |
PFCE | Dye | Rodent glioma | 0.2 | 1 | [34] | |
PFOB | Drug | 225 | Primary human umbilical cord smooth muscle cells | Multiple | [35] | |
Various PFCs | Fluorinated quantum dots | 200 | Murine macrophage Human carcinoma | Multiple | [36] | |
PFCE | Targeting peptide | 170 | Human umbilical cord vein Endothelial cells | 1 | [37] | |
PFOB | Targeting antibody Transfection agent | Human cancer cell lines | Multiple | |||
PFCE | Dye | 145 | Macrophages | 1 | [40] | |
Hyperbranched fluorinated polymer | Drug | 20–30 | Human glioblastoma cell line | 0.0005 | 1 | [41] |
PERFECTA | n/a | 140–220 | Dendritic cells | 19.2 | 1 | [14] |
Fluorinated colchicine derivatives | n/a | n/a | Human leukemia cell line | Multiple | [42] | |
Fluorinated reporter molecules | n/a | n/a | Various, including bacteria and human cancer cell lines | |||
Fluorinated prodrug | Human cancer cell lines | [46] |
There have been limited studies using fluorinated drugs (see Table 1). The limit here is the high reactivity and electronegativity of fluorine, which when incorporated into a drug can significantly alter drug chemistry and subsequent metabolism. Chemical shift artifacts can also be an issue. For all these reasons, such small molecules are seldom used for cell tracking.
2.3 Perfluorocarbons
Perfluorocarbons (PFCs) are synthetic molecules mainly composed of carbon and fluorine atoms (see Table 2 for a summary of their physical properties). Depending on their chemical structure and molecular weight, PFCs exist in a gaseous, liquid, or even solid form. The compounds are extremely stable, generally have low vapor pressures, and are inert. PFCs do not react with living tissues due to their carbon-fluorine bonds, which cannot be metabolized in vivo, and they have low intermolecular forces, and hence, the surface tension of the liquid form of PFCs is extremely low. Most PFCs have a surface tension of 14–18 dyne/cm [9]. As gases, PFCs show some toxicity, while in a liquid form they are relatively safe [10]. Clearance of PFC agents from the body generally occurs via the mononuclear phagocyte system (MPS) uptake (phagocytic cells primarily in lymph nodes, spleen, and liver) and finally lung exhalation. Some PFCs can also bind and dissolve oxygen. This led to their early use as blood substitutes, although the idea has been commercially abandoned. PFCs are still used clinically, for example for partial liquid ventilation [LiquiVent, Alliance Pharmaceutical Corporation], eye surgery [Perfluoron™, Alcon® Inc., USA], and as ultrasound contrast agents as gas bubbles [Sonovue®, Bracco International B.V.] (Fig. 2a).
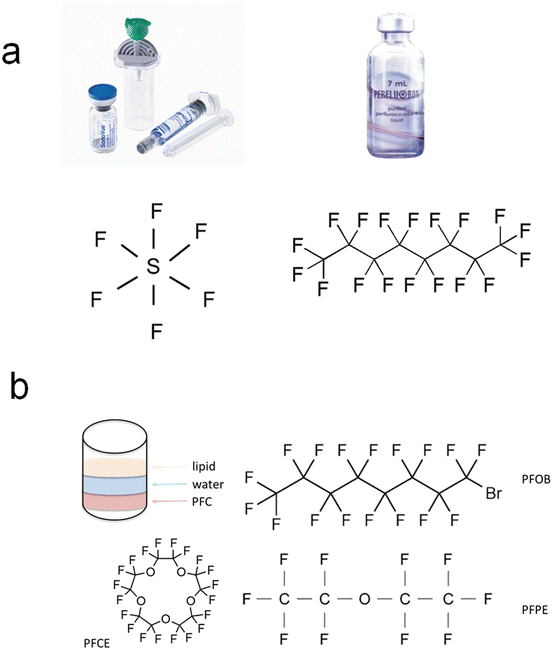
Table 2
General physical properties of various PFCs used in cell tracking
Perfluorocarbon | Formula | Molecular weight | Density (g/mL) | Boiling point (°C) |
---|---|---|---|---|
Perfluoropentane | C5F12 | 288 | 1.62 | 26–36 |
Perfluorohexane | C6F14 | 338 | 1.76 | 58–60 |
Perfluorooctyl bromide | C8F17Br | 499 | 1.93 | 140.5 |
Perfluorononane | C9F20 | 488 | 1.80 | 126 |
Perfluorodecalin | C10F18 | 462 | 1.99 | 144 |
Perfluoro-15-crown-5-ether | C10F20O5 | 580 | 1.78 | 145 |
PERFECTA | C21H8F36O4 | 1008 | 2.09 | ND |
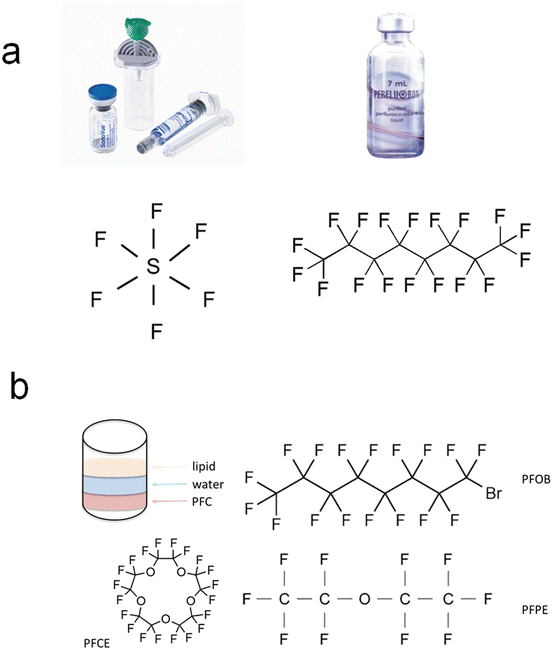
Fig. 2
Perfluorocarbons. (a) PFCs are currently in clinical use, as both gases and liquids. Shown is an ultrasound microbubble contrast agent containing sulfur hexafluoride gas (Sonovue®) and a perfluorooctane liquid used in eye surgery (Perfluoron™). (b) Most cell labels are perfluorocarbons (PFCs), polymers of carbon and fluorine. These compounds have unique properties; in particular they mix with neither aqueous nor organic solvents. Some of the more commonly used PFCs for cell tracking are PFOB, PFCE, and PFPE, shown here
PFCs have now found their use as imaging labels for ultrasonography, computed tomography, and 19F MRI. Their use in the latter imaging method is especially beneficial, as PFCs have a high payload of fluorine atoms, which makes 19F MRI a promising modality for in vivo quantitative imaging, including in vivo cell tracking. Here, liquid PFCs are more suitable, as they result in the most favorable imaging characteristics.
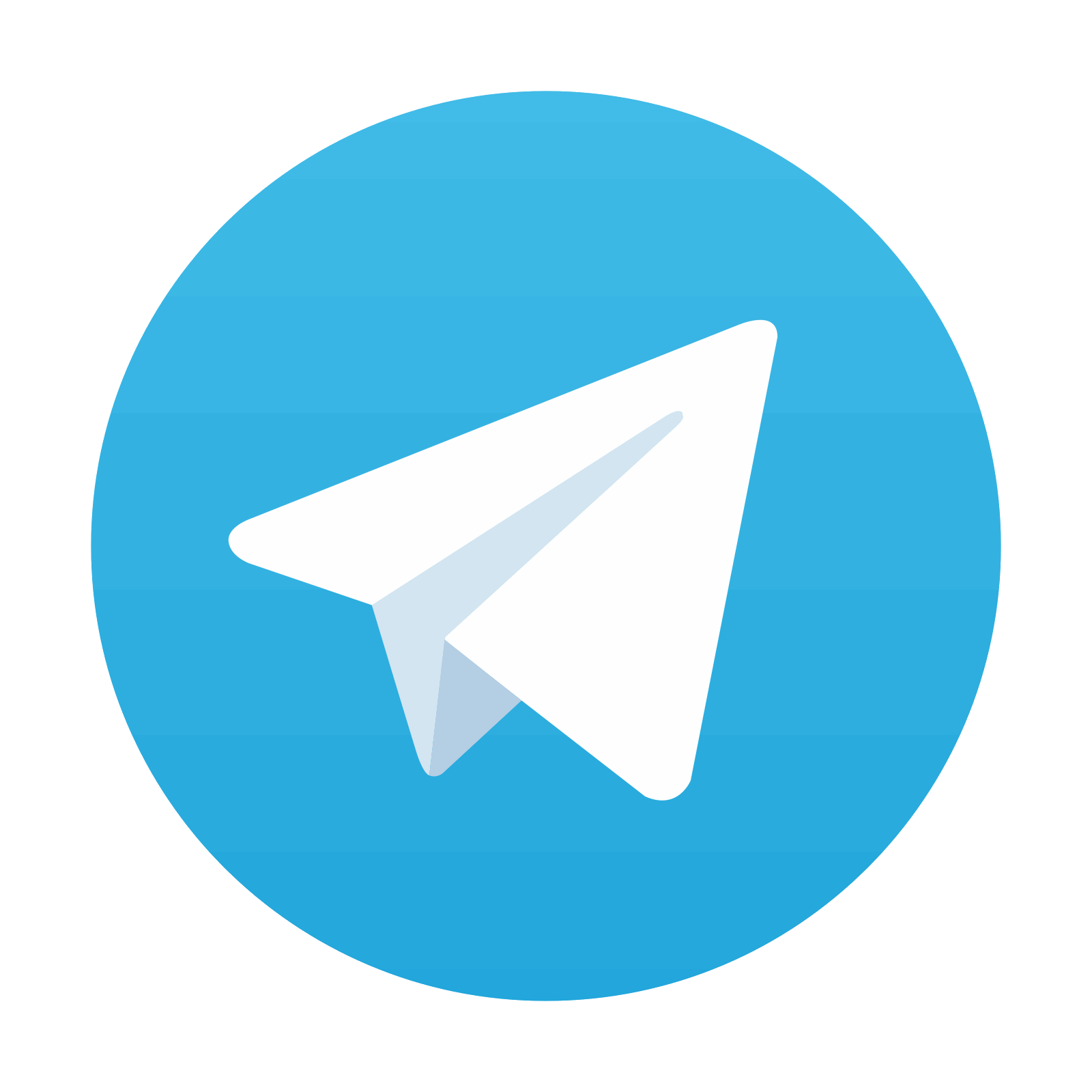
Stay updated, free articles. Join our Telegram channel

Full access? Get Clinical Tree
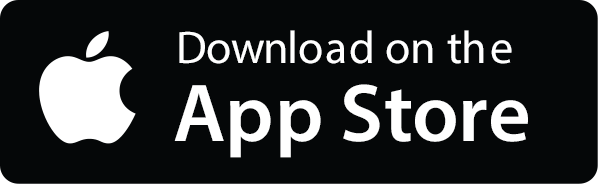
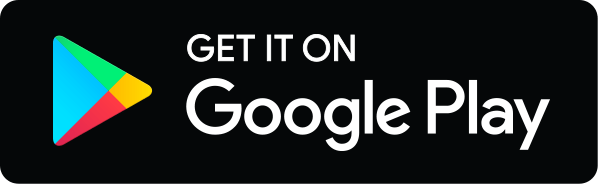