FIG. 15.1 A: AP oblique right vertebral arteriogram in the late arterial phase demonstrates the anterior spinal artery (ASA) (small arrow) descending from the proximal basilar artery. Note the ASA in this patient divides into two branches (large arrows). B: Lateral right vertebral arteriogram in the late arterial phase again demonstrates the ASA arising from the basilar artery (small black arrow) and dividing into two branches (large arrows) in the upper cervical region. Note the dissecting vertebral artery aneurysm just proximal to the origin of the ASA (white arrow).
Similarly, the paired posterior spinal artery (PSA) is formed from branches of the intracranial vertebral or posteroinferior cerebellar arteries. The PSAs extend caudally along the posterior surface of the spinal cord just off midline, and together, they supply the remaining one-third of the spinal cord. The PSA branches are centripetal and supply the dorsal 30% of the cord. The deep arterial (or intrinsic) system is located within the substance of the cord itself and is comprised of the perforating sulcal (central) and radial (peripheral) arteries. There are no substantial anastomoses between the ASA and PSAs, and thus, the watershed territory of the cord is located at the periphery of the gray matter (2).
The ASA and PSAs receive various contributions from segmental spinal arteries that enter the spinal canal as medullary or radicular arteries via the neural foramina (1–4). They may arise from branches of the subclavian artery (ascending cervical artery, deep cervical artery) and branches of the aorta (supreme intercostal artery, posterior intercostal arteries, lumbar radicular arteries, and lateral and middle sacral arteries) (3). The radicular arteries supply three separate functional regions of the spinal cord from C1 to T2, from T3 to T7, and from T8 to the conus medullaris (4). The midthoracic spinal cord from T3 to T7 receives fewer radicular arterial contributions, in part due to a greater distance between radicular arteries; as a major watershed area, it is particularly vulnerable to cord infarction (5). The artery of Adamkiewicz is the largest of the anterior radicular arteries and provides the primary supply to the ASA in the lower thoracic and lumbar spine (region of spinal cord lumbar enlargement). It is most commonly located on the left arising from an intercostal or radicular artery, entering the spinal canal between the levels of T9 and L1, and makes a characteristic hairpin loop (Fig. 15.2).
FIG. 15.2 Artery of Adamkiewicz. A, B: AP and lateral DSA, respectively, demonstrate the artery of Adamkiewicz (arrow) arising from the right T9 radicular artery with its characteristic hairpin loop. C: Dual-energy CTA volumetric curved reformat, in a different patient, also demonstrates the artery of Adamkiewicz (arrow).
The venous anatomy of the spine and spinal cord is highly variable but can also be divided into intrinsic and extrinsic systems (1,2,6). The intrinsic system is comprised of the sulcal and radial veins that drain the center and periphery of the spinal cord, respectively. These intrinsic veins eventually drain into the extrinsic system or superficial veins that run along the pial surface of the cord; this system is called the coronal venous plexus and is comprised of anterior and posterior spinal veins. Unlike their arterial counterparts, the superficial veins form a rich collateral network. The anterior and posterior spinal veins then drain into their respective radicular veins, which travel to the epidural space to empty into Batson plexus, an intercommunicating venous network comprised of the internal vertebral plexus, the external vertebral plexus, and the basivertebral veins (6).
Imaging Choices, Limitations, and Protocols
Plain film radiography and myelography
Historically, imaging of the spine was limited to plain film radiography, which is useful for evaluating the vertebral bodies but otherwise has no role in the evaluation of the spinal cord and vasculature. Rarely, a large mass in the spinal canal or dural ectasia may result in erosion of the spine that can be identified on plain film radiography.
The first attempt to image spinal cord pathology commenced with myelography, requiring a lumbar puncture and administration of iodinated intrathecal contrast (7). The earliest contrast agent was Pantopaque (iodophenylundecylic acid), an oily iodinated contrast media introduced intrathecally and removed at the end of the examination. Although Pantopaque was first replaced by water-soluble iodinated ionic contrast (metrizamide) that did not require removal and more recently with even safer, water-soluble nonionic contrast (iohexol), we occasionally see a CT or MRI with residual oily media in the subarachnoid space (Fig. 15.3). On myelography, the spinal cord is seen in relief, surrounded by dense contrast within the spinal subarachnoid space. Intramedullary (within the cord); intradural, extramedullary; and extradural lesions are delineated by distortions of the opacified spinal subarachnoid space (7). Mass lesions, such as drop metastases from primary brain tumors, manifest as filling defects within the contrast column, while enlargement or engorgement of vascular structures manifests as tubular filling defects. CT following the administration of water-soluble intrathecal contrast agent (CT myelogram) enhances the evaluation as it demonstrates the relationships of the thecal sac and nerve root sleeves to the bony vertebral column, epidural fat, and intervertebral disks. Myelography is currently employed when there are contraindications to the use of MRI, such as MRI incompatible cardiac pacemakers or other metallic foreign bodies that might make MRI imaging either unsafe or suboptimal in quality (8).
FIG. 15.3 Pantopaque myelography and MRI. A,B: Contrast enhanced sagittal and axial T1 weighted images demonstrate punctate T1 hyperintense foci (arrows), suspected to be enhancing vessels associated with a spinal dural arteriovenous fistula. C: Axial CT myelogram image demonstrates nonionic contrast within the thecal sac as well as more hyperdense discrete droplets of Pantopaque oily contrast from past myelography in this 61-year-old patient with myelopathy.
Spinal digital subtraction (catheter) angiography
Diagnostic DSA is a dynamic, catheter-based examination that offers anatomic and functional evaluation of the spinal circulation with excellent spatial and temporal resolution. DSA has supplanted “cut-film” angiography with its improved resolution and detail. Acquisition of DSA images entails capturing a “mask” image prior to the injection of contrast, which is then digitally subtracted from postcontrast injection images in order to reveal the vasculature without the bone and soft tissue. The transit of contrast through the arteries, capillaries, and veins corresponds to their three distinct angiographic phases (9). Digital rotational angiography with 3D reconstruction aids in analysis of aneurysms and arteriovenous malformations (AVMs) involving the spinal vasculature (10).
Complete, catheter-based, spinal arteriography is invasive and time consuming and may require large amounts of contrast and radiation (11). Intra-arterial access is achieved by catheterization of the common femoral artery with placement of a short arterial sheath and a Foley catheter to prevent an enlarged, contrast-filled bladder from obscuring lumbosacral spinal arterial anatomy and pathology. Careful interrogation of the spinal vasculature requires selective catheterization of each spinal radicular artery and digital filming, often with general anesthesia and suspended respiration for the brief filming period to minimize respiratory motion and improve conspicuity of tiny spinal arterial branches. The examination is not complete without catheterization of the middle sacral artery (arising from the aortic bifurcation) and the hypogastric (internal iliac) arteries to assess the most caudal spinal vascular contributions. Within the cervical region, vertebral arteries, subclavian artery branches (including the ascending cervical, deep cervical and thyrocervical trunk), and several external carotid artery branches (particularly the occipital and ascending pharyngeal arteries) may also contribute to the vascular supply of the spine and spinal cord.
Catheter-based spinal angiography is the reference standard for the diagnosis of spinal vascular malformations, including dural arteriovenous fistulae, and localization of the artery of Adamkiewicz prior to aortic or scoliosis surgery (12). Both conditions distort the aortic anatomy and the origins of the radicular arteries, making catheterization more difficult (Fig. 15.4). Catheter-based spinal angiography remains an important diagnostic examination in the workup and treatment of vascular vertebral masses. Many such lesions may require embolization prior to or in lieu of surgical excision.
FIG. 15.4 Scoliosis and spinal DSA. A: Scoliosis with hemivertebra and apex at the thoracolumbar junction often requires DSA for localization of the ASA prior to corrective surgery. B: Catheter in the radicular artery of T9 on the left demonstrates the artery of Adamkiewicz (AA, arrow).
Spinal DSA is not without its risks and complications as it carries a 0.4% to 1% risk of spinal cord ischemia and infarction (11,12). Additional considerations include radiation exposure, contrast-induced nephropathy, hematomas at the access site, and infection. As for any procedure, invasive or noninvasive, the benefits must be weighed against potential risks.
Computed tomographic angiography (CTA)
Spinal CT angiography (CTA) is a rapid and minimally invasive imaging modality that provides high-resolution and high contrast arterial opacification in order to delineate the spinal arterial vasculature, namely, the artery of Adamkiewicz, and to characterize spinal vascular malformations (13,14). After injection of a contrast bolus at a rate of at least 3.5 mL/s, images are helically acquired and reconstructed in 0.625-mm slice thickness at no greater than 0.25-mm slice intervals. Multiple intensity projection (MIP) images, curved reformations, or volume-rendered images may be helpful for diagnosis (14,15). Dual-energy CTA techniques may be employed with increased resolution and conspicuity of the artery of Adamkiewicz. Use of sublingual nitroglycerin approximately 10 to 20 minutes prior to imaging will dilate the spinal vasculature and increase the conspicuity of the ASA (analogous to protocols used in cardiac CTA) (Fig. 15.5). Limitations of CTA include the use of iodinated contrast (which can preclude its use in patients with contrast allergies), the risk of contrast-induced nephropathy, failure to obtain optimal vessel opacification related to a patient’s comorbidities or technical difficulties, time and skill required for postprocessing, and the potential for image distortion during reconstruction from beam hardening artifact originating from dense bone (13–15).
FIG. 15.5 Dual-energy CTA. A,B: Sagittal and coronal curved reformat CTA reconstructions, respectively, reveal multiple tortuous vessels on the posterior and left lateral surface of the spinal cord spanning multiple spinal levels, consistent with dural arteriovenous fistula (DAVF).
MRI/MRA
MRI, with its multiplanar capabilities and high soft tissue detail, is the diagnostic examination of choice for the evaluation of spinal pathology. For practical purposes, MRI is most useful for visualization of the spinal cord within the spinal canal. MRI sequences can be tailored to investigate specific clinical concerns (16). Fast spin-echo (FSE) T2-weighted sequences are used to evaluate the spinal cord and nerve roots. In fast spin-echo inversion recovery (FSE-IR) or short tau inversion recovery (STIR) imaging, T2-weighted images are obtained with concurrent suppression of signal from fat. This is particularly useful to assess for bone marrow edema or to accentuate the conspicuity of intrinsic cord lesions. T1-weighted sequences are routinely used for the evaluation of bone marrow. Proton density-weighted imaging is frequently used to assess intrinsic cord signal abnormality. Gradient recalled echo (GRE) imaging serves to survey spinal canal contents for hemorrhage or hemosiderin deposition in the setting of tumor or vascular lesions. Intravenous contrast administration of gadolinium, similar to CT iodinated contrast, is helpful in situations of increased vascular perfusion and “blood–brain barrier” breakdown as seen in infection, neoplasm, vascular malformation, or leptomeningeal disease (Fig. 15.6) (17).
FIG. 15.6 Value of contrast MR—conus paraganglioma. A,B: Pre- and postcontrast T1-weighted sagittal images, respectively, demonstrate an avidly enhancing, lobulated mass causing scalloping of the vertebral bodies from L3 through S1. C: The expansion of the spinal canal by the mass and the scalloping is well seen on the post contrast axial T1 weighted image. D: Midline sagittal T2-weighted image demonstrates marked abnormal vasculature surrounding the conus. E: DSA demonstrates the artery of Adamkiewicz at the right T9 level supplying the large dilated arterial supply (arrow) to the tumor.
Spine MR angiography (MRA) remains a noninvasive alternative to DSA. It is implemented utilizing a three-dimensional, contrast-enhanced, gradient-echo technique (18). Postprocessing is performed using multiplanar maximum intensity projections (Fig. 15.7). Applications of spinal MRA include preoperative localization of the artery of Adamkiewicz and detection of feeding vessels in AVMs and dural fistulae, and spinal MRA has been favorably compared to spinal CTA (19,20). Limitations include the inability to resolve small-caliber vessels, which necessitates the use of conventional catheter angiography.
FIG. 15.7 Spinal MRA. Postcontrast spinal MRA MIP image demonstrates arteries (white arrows) and veins (black arrows) on the same image indicating the presence of arteriovenous shunting in this patient with a large metameric spinal arteriovenous malformation.
Diffusion-weighted imaging (DWI) is based on the random movement of water molecules by Brownian motion and provides physiologic information about imaged tissue. This technique has been successfully employed in the brain for the diagnosis of cerebral infarction and is becoming increasingly utilized for the evaluation of spinal cord infarction. It can play a vital role in the detection of hyperacute cord infarction in which cytotoxic edema may not be detectable by conventional T2-weighted imaging (21). Acquisition of diffusion-weighted images of the spine can be technically challenging due to the inherent small size of the spinal cord, susceptibility artifact from the bony spinal canal, and flow/pulsation artifact from cerebrospinal fluid and spinal arteries (22).
Spinal Vascular Lesions
Spinal vascular lesions can be broadly divided into lesions with increased vascularity such as spinal vascular malformations and hypervascular tumors and lesions with decreased or impaired vascularity leading to ischemia and infarction. Although the cavernous malformation has a venous vascular origin, it behaves like a mass lesion within the spinal cord with a distinctive imaging appearance. Traumatic injury to the vertebral or radicular arteries may occur as a consequence of blunt or penetrating trauma or secondary to iatrogenic interventions (Table 15.1).
Table 15.1 Spinal Vascular Lesions—Broad Categorization
Spinal vascular malformations
Spinal vascular malformations include AVMs, arteriovenous fistulae (pial, dural, or mixed—AVF, DAVF), and cavernous malformations (also known as “cavernomas”). Capillary malformations have not been described in the spinal cord. Categorization schemes relate to the anatomic location and appearance of the AVM or AVF (Table 15.2) (22–24). MRI is the first-line screening modality for spinal vascular malformations and may guide further imaging, management, and treatment (25).
Dural AVF/type I spinal arteriovenous malformation
The dural arteriovenous fistula (DAVF, DAVM, dural arteriovenous malformation) is the most common spinal vascular malformation (23,24). DAVF is felt to be acquired, is usually located in the thoracolumbar region, and usually occurs in middle-aged males (23,24). DAVF is composed of direct communications between the arteries and veins without an interposing nidus. The fistulous communication is located within the dura of a proximal nerve root sleeve (26). The arterial inflow to the fistula arises from a radiculomeningeal artery, while the venous drainage is intradural, into the medullary veins that normally provide the venous drainage for the spinal cord. Spinal DAVF frequently have a single feeding artery, but may also have multiple feeding arteries. It is important to recognize additional vessels for treatment purposes. The vein drains intradurally connecting with and transmitting the increased pressure to the coronal venous plexus. This results in dilated veins that often extend cranially and caudally from the site of the fistula along the dorsal aspect of the cord. The intradural location of the draining veins and lack of venous valves lead to spinal venous hypertension, which induces venous congestion and edema of the spinal cord. There is interference of the venous drainage from the spinal cord from high-pressure arterial flow within the fistula with associated decrease in arteriovenous pressure gradient and chronic cord hypoxia. Patients with DAVF often have a long, insidious clinical course of progressive myelopathy and potentially irreversible neurologic deficits (27,28).
Imaging Findings
MRI has become extremely useful in identifying the presence of DAVF and for guiding angiography and therapy (Fig. 15.8). MRI often demonstrates a combination of cord edema and perimedullary dilated veins (25,29–31). The most sensitive MRI finding is the presence of cord edema characterized by T2 hyperintense signal within the cord parenchyma that may extend over multiple vertebral levels. The conus is involved in up to 87% of cases (29–31). Postcontrast images can show dilated, tortuous vessels on the surface of the cord as well as patchy enhancement of the spinal cord. Venous congestion can also result in cord enlargement in up to 45% of cases (29–31). In a study of 20 patients, Saraf-Lavi et al. demonstrated a sensitivity of 85% to 90% and a specificity of 82% to 100% in detection of DAVF using MRI and MRA (29). Intradural serpentine enhancement that extended greater than 3 vertebral levels was most frequently associated with DAVF. The detection of flow voids on T2-weighted images and the presence of more than two major vessels on the cord surface on MRA were each strongly associated with DAVF (29).
FIG. 15.8 Type 1 spinal arteriovenous malformation, DAVF. A: Sagittal T2-weighted image demonstrates increased signal within the lower spinal cord and conus medullaris with multiple enlarged tortuous vessels dorsal to the spinal cord. B: Parasagittal T2-weighted image demonstrates focal hypointensity within the cord indicative of hemorrhage (arrow). C: Postcontrast T1-weighted image reveals multiple enhancing vascular structures along the posterior surface of the spinal cord representing dilated draining veins. There are also enhancing foci within the spinal cord.
CTA or MRA can also help to direct catheter angiography by identification of the segmental arterial involvement and potentially limiting the number of radicular arteries to be injected to completely identify the feeding arteries prior to treatment (15). DSA is the reference standard for diagnosis and mapping of the spinal DAVF to guide treatment (Fig. 15.9). These lesions may be treated with open surgery by identification and clipping of the fistula adjacent to the nerve root (30). Endovascular treatment by embolization of the fistula can be performed and may be used as a presurgical adjunct or as definitive treatment in a minority of cases (31,32).
FIG. 15.9 Type 1 spinal dural arteriovenous fistula. A: Sagittal T2-weighted image shows T2 hyperintensity within the conus medullaris and the lower thoracic spinal cord. Multiple flow voids are noted along the posterior aspect of the spinal cord with tortuous vessels in the region of the cauda equina (arrow). B, C: AP and lateral DSA images demonstrate the fistula (artery to venous connection; arrows) and multiple draining veins responsible for the venous congestion and clinical symptoms of leg weakness. D: Following surgical treatment, the patient’s clinical symptoms improved and a sagittal T2-weighted image reveals resolution of the abnormal T2 hyperintensity within the spinal cord and of the vascular flow voids along the dorsal surface of the cord.
After treatment of spinal DAVF, many of the characteristic MRI findings resolve or diminish, including spinal cord edema and dilated subarachnoid vessels. Enhancement of the cord may persist as a result of irreversible breakdown of the blood–cord barrier from chronic ischemia, even if T2 signal abnormality resolves. Kaufmann et al., in an analysis of 34 patients with DAVF, found MR abnormalities to persist after treatment in 91% (31/32) of patients) (33). T2 signal abnormality may also persist, if chronic hypoxia is severe enough to cause permanent cord injury with gliosis.
Extradural arteriovenous fistulae
Extradural arteriovenous fistulae are usually supplied by a branch of a radicular artery that directly communicates with the epidural venous plexus. They result in significant enlargement of the epidural venous system. The dilated veins and/or an associated epidural hematoma can cause mass effect on the nerve roots and the spinal cord. A unique form of extradural arteriovenous fistula can arise spontaneously in the setting of neurofibromatosis type I (NF-I) (Fig. 15.10) (34). This form occurs in the cervical region with fistulae between the vertebral artery and a large intraspinal venous varix with drainage into the extradural and the paraspinal venous plexus. Affected patients have the stigmata of NF-1 and cervical bruit and may develop a variety of neurologic findings related to compressive myelopathy. These fistulae do not drain intradurally and therefore do not cause venous congestion within the cord as is seen with DAVF type I.
FIG. 15.10 Vertebral extradural fistula in neurofibromatosis type 1. A: Midline sagittal T2-weighted MR image demonstrates a large extradural vascular flow void, consistent with a dilated venous structure ventral to the spinal cord. B: Sagittal T2-weighted image to the left of midline demonstrates a large paraspinal vein (arrow). C: Findings are confirmed on the axial T2-weighted image, which also demonstrates enlargement of the neural foramen on the left secondary to pressure effect from the vessels. Note the signal intensity of these flow voids is the same as the great vessels (arrow). D: Right vertebral DSA demonstrates steal to the fistula on the left and best demonstrates the location of the fistula (arrow). E: AP left vertebral DSA demonstrates the enlarged proximal vertebral artery (arrows) and the patulous extradural venous pouch (EV) as well as the enlarged cervical veins. (Courtesy of Cliff Eskey, MD.)
Spinal arteriovenous malformations types II to IV
True AVMs are congenital spinal vascular abnormalities composed of abnormal communication of arteries to veins without an intervening capillary network. They can be divided into glomerular, perimedullary, and juvenile types (35).
Type II spinal arteriovenous malformations: glomerular
The glomerular type is the most common spinal cord AVM (type II AVM or angioma racemosum) and has a well-defined intramedullary nidus with or without a superficial perimedullary component (23,35). The nidus can be compact or diffuse. Type II AVMs are supplied by multiple radiculomedullary branches of the ASA and PSA and are characterized by high pressure and high flow related to arteriovenous shunting into low-resistance veins. The high pressure predisposes to aneurysm formation, which is seen in up to 50% of cases. High pressures also result in venous varices that can compress the cord. They can occur anywhere in the spinal cord, but are somewhat more common in the cervicothoracic region.
Type II AVMs have no gender predilection and tend to present at a younger age than do DAVF. They tend to have more sudden onset of symptoms, often as a result of subarachnoid or intramedullary hemorrhage, presenting with acute back pain or acute myelopathy due to compression. A more gradual progressive myelopathy may result from arterial steal, arteriovenous shunting, venous hypertension, or compression from spinal aneurysms or venous varices.
Imaging Findings
MRI reveals the nidus as an intramedullary (with or without perimedullary) tangle of dilated flow voids on T2-weighted images (Fig. 15.11) (8,16,25). T1-weighted images reveal hyperintense and hypointense tubular structures depending on the flow velocity and direction. Enlarged dorsal vessels may compress the spinal cord. The spinal cord may be focally expanded by the mass effect from the nidus. Hyperintense signal within the cord parenchyma on T2-weighted imaging adjacent to the nidus may represent cord edema, infarction, and/or gliosis. Hemosiderin from prior hemorrhage causes hypointensity on GRE magnetic susceptibility imaging. On DSA, the arterial supply is from the ASA more often than the posterior spinal arteries (10,23,24). There are usually multiple feeding arteries. Venous drainage is via intramedullary and perimedullary veins into the coronal venous plexus. Type II AVMs have high pressure and high flow, with rapid filling and early venous drainage demonstrated on DSA.
FIG. 15.11 Type 2 spinal arteriovenous malformation, glomus type. A: Sagittal T2-weighted MR image demonstrates an intramedullary nidus composed of multiple flow voids. Note the increased signal in the adjacent cord above the lesion and atrophy (arrow) below the lesion. B: Sagittal GRE image reveals intramedullary susceptibility consistent with blood products.
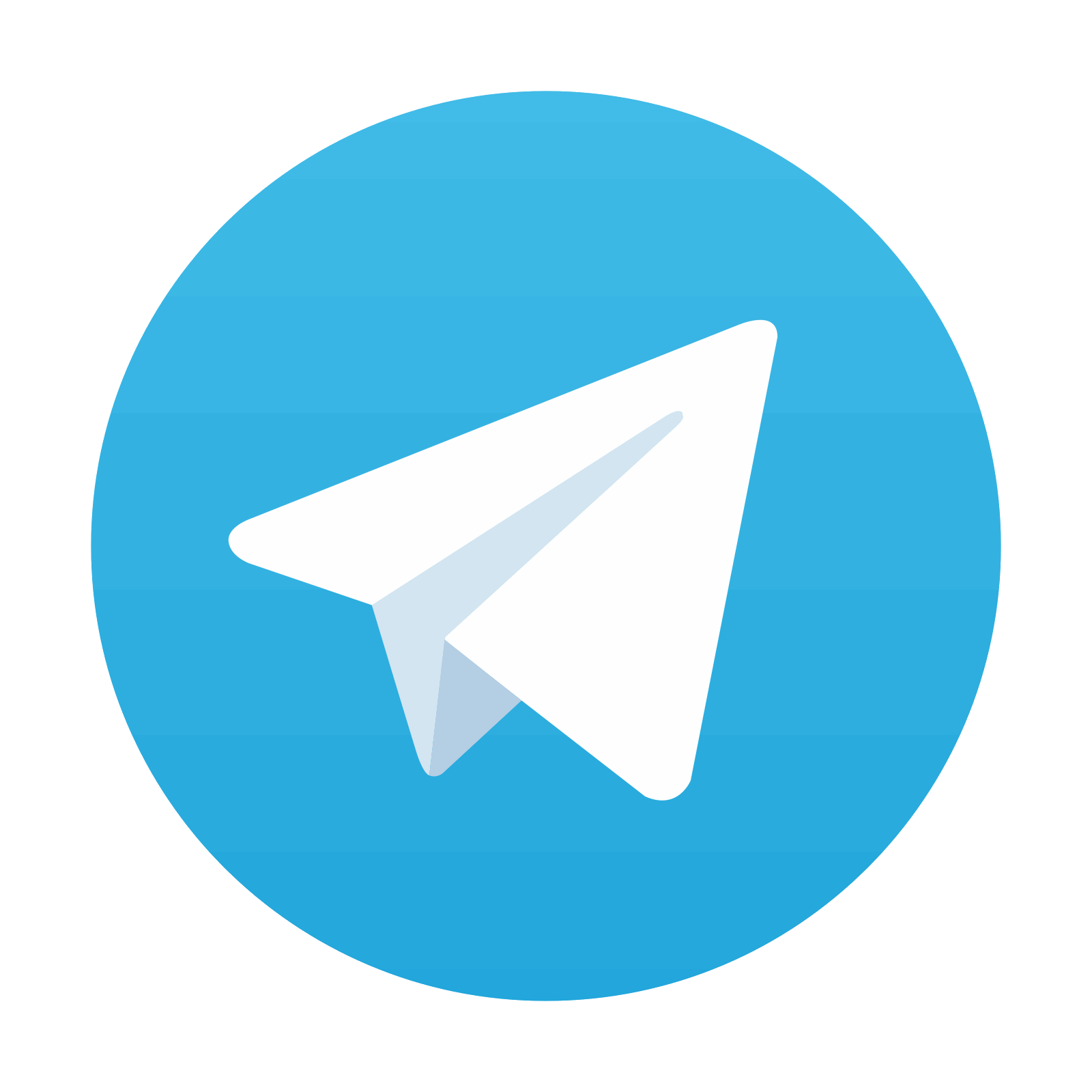
Stay updated, free articles. Join our Telegram channel

Full access? Get Clinical Tree
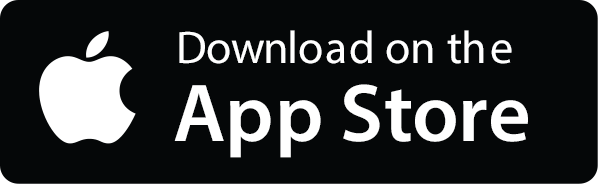
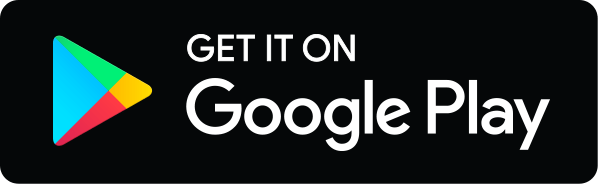