Ahmad Shariftabrizi1,2, Khalid Balawi1, and Janet H. Pollard1 1 University of Iowa Carver College of Medicine, Iowa City, IA, USA 2 Veterans Affair Medical Center, Iowa City, IA, USA Nuclear medicine offers an array of methods for imaging the pathophysiology of diseases affecting the vascular system. The spectrum of vascular disorders ranges from acute, life‐threatening situations to chronic processes, and spans the entities of infection, inflammation, trauma, and oncologic pathologies. Diagnostic imaging of the vascular system is dominated by ultrasound (US), computed tomography (CT), magnetic resonance imaging (MRI), and conventional angiography. Nuclear medicine with planar imaging, single‐photon emission computed tomography (SPECT), and positron emission tomography (PET) provides important qualitative and quantitative information about biologic processes, as well as dynamic information about vascular flow, tissue perfusion, and trends in tissue uptake of radiotracer. These modalities are highly sensitive and able to detect minute quantities of radiotracer in the nano‐ to picomolar range. Nuclear imaging may detect disease processes before morphologic changes are evident on other imaging modalities, allowing for earlier opportunities for therapy in some cases. PET and non‐PET radionuclides differ in their production methods and decay times. Fluorinated PET radiopharmaceuticals such fluorine‐18 (18F)‐fluorodeoxyglucose (18F‐FDG) and 18F‐sodium fluoride (18F‐NaF) require cyclotron isotope production. Given the relatively short half‐life of 18F (110 minutes), imaging centers must be within an accessible distance of radiopharmacies with cyclotrons. The longer half‐lives of the non‐PET radiotracers such as technetium‐99 m (6 hours) and indium‐111 (67 hours) offer greater flexibility in availability, timing, and coordination. Compared to planar and SPECT imaging, PET has the advantages of a higher limit of resolution (2 mm vs. 10 mm), attenuation correction, and quantitative capability [1]. At this time hybrid PET/CT systems are in widespread clinical practice. Hybrid SPECT/CT systems are also widely available. Hybrid PET/MRI systems are of growing interest in research and clinical settings, but their availability is still limited. In this chapter cases of vascular pathology with correlative nuclear and anatomic imaging will be discussed. Areas covered include inflammation, infection, neoplasia, molecular receptor expression, and physiologic processes such as osteogenesis. Vasculitis is a heterogeneous group of multisystem diseases characterized by inflammation of the blood vessels. Noninfectious vasculitides are classified based on vessel size into categories of large, medium, and small vessel vasculitides (SVV). Vasculitides usually affect vessels in their respective category for size, however, any size vessel can be affected. For example, large vessel vasculitis affects the large vessels more often than other vasculitides. Examples include the large vessel vasculitides (LVV) Takayasu arteritis (TAK) and giant cell arteritis (GCA), the medium vessel vasculitides (MVV) polyarteritis nodosa (PAN) and Kawasaki disease, and the SVV granulomatosis with polyangiitis (GPA) (also known as Wegener’s), pauci‐immune antineutrophil cytoplasmic antibody (ANCA)‐associated vasculitis, and immune complex small vessel vasculitis [2]. Imaging of vasculitis includes US, CT, MRI, and MRI angiography (MRA), conventional catheter angiography, and nuclear imaging. US is a targeted imaging modality with a limited field of view that measures intima‐media thickness and luminal changes in large and medium‐sized vessels. Contrast‐enhanced CT (CECT) offers greater anatomic coverage with excellent spatial resolution and multiplanar and three‐dimensional viewing capabilities. CT can show changes in thickness of the vessel wall and lesion extent. CT also offers more global assessment of various organs, which can help with differential diagnosis and complications. MRI/MRA can identify vessel abnormalities such as wall edema, mural enhancement, and luminal changes, and can detect early wall thickening even before luminal narrowing occurs [3]. Conventional angiography detects later stages of disease after stenosis or other luminal abnormalities have developed. As a whole‐body imaging modality, nuclear imaging can visualize disease distribution in various anatomic regions simultaneously. In certain types of vasculitis, 18F‐FDG PET uptake correlates with disease severity and has a role in the detection of disease, assessment of extent of disease, and noninvasive monitoring of response to treatment for some types of vasculitis [4]. 18F‐FDG PET has a limited role in the direct detection and management of small vessel vasculitis but can aid in the diagnosis of systemic manifestations and direct biopsy. Technical factors and patient preparation can affect the ability to detect vasculitis. Compared to other imaging modalities, FDG PET/CT gives limited anatomic information about the vessel lumen (e.g. stenosis, occlusion, or aneurysm) and has limited resolution in small vessels. Although high‐resolution PET scanners are available that can detect vascular inflammation in relatively small arteries, such as the temporal, facial, and maxillary arteries, clinical availability is variable [5]. Additional challenges with FDG PET are interference from glucocorticoids and need for controlled serum glucose. FDG PET should be performed before or no more than 3 days after initiation of glucocorticoid therapy. Sensitivity declines to one‐third after 10 days of glucocorticoid therapy. Alternatively, steroids should be discontinued for at least 2 weeks prior to FDG PET if the patient is treated with >10 mg per day [4]. Technical parameters such as uptake time can affect the sensitivity of FDG PET as well. Image acquisition at 120 minutes rather than 60 minutes post‐injection improves the target‐to‐background of vascular wall uptake [5] (Figure 9.1). FDG uptake in the aorta and branch vessels that is equal to or greater than the liver background, or in the extracranial vessels of the head and neck that is greater than adjacent tissue, is suggestive of vasculitis. High FDG uptake in a continuous linear or homogenous segmental and often circumferential pattern is consistent with vasculitis. False positives can occur with atherosclerosis. Although the uptake patterns can overlap, in atherosclerosis uptake tends to be inhomogeneous, focal, or patchy with overall milder intensity compared to vasculitis. With higher resolution PET scanners, inflammation can be detected in the small cranial arteries such as temporal, facial, and maxillary arteries (Figure 9.2). PET can detect pathologic mimics of vasculitis such as infection, malignancy, and atherosclerosis. High FDG uptake in a homogenous segmental and often circumferential pattern is consistent with LVV. Atherosclerosis also causes FDG uptake and should be distinguished from LVV. However, atherosclerosis often has milder and more inhomogeneous focal or patchy uptake compared to LVV, which usually appears as intense, smooth linear often circumferential uptake (Figure 9.3). Although the two patterns can overlap, FDG uptake is usually symmetric in GCA. PET shows greater sensitivity for inflammation than MRI and can be used for disease monitoring [5]. Disadvantages of PET/CT include lack of anatomical information about luminal changes, radiation exposure, the need to be relatively free of glucocorticoids, and the need for optimal glucose concentration [5, 6]. Figure 9.1 Changes in aortic wall and luminal blood FDG activity at different imaging time points as seen on sagittal FDG PET images of the thoracic aorta. With time, luminal blood activity decreases while the aortic wall activity increases, which improves the arterial wall‐to‐blood contrast (superior target‐to‐back ground ratio). (Source: Reproduced from: Moghbel et al. [27].) Figure 9.2 The 18F‐FDG PET/CT maximum intensity projection images of GCA patients. (A) Large vessel‐giant cell arteritis (LV‐GCA) with increased FDG uptake consistent with vasculitis in aorta, subclavian, axillary, and vertebral arteries. In the lower limb arteries, FDG intensity is not higher than liver FDG uptake and can be due to either vasculitis or arteriosclerosis. (B) Vertebral artery high‐intensity FDG uptake in a GCA patient. (C) A GCA patient with widespread cranial artery involvement. Increased FDG uptake higher than surrounding tissue is recognized in temporal, maxillary, occipital, internal, and external carotid artery. (Source: Reproduced from: Schmidt and Nielsen [5].) US is currently the recommended modality for the initial diagnosis of GCA. A standard US examination in GCA should include temporal and axillary arteries. Measuring the intima‐media thickness in the temporal and axillary arteries allows rapid diagnosis of GCA, with reported sensitivity and specificity of 77% and 96%, respectively. The European League Against Rheumatism (EULAR) recommends that if US is unavailable or inconclusive, MRI of the cranial arteries maybe used. In a recent metanalysis of six studies, the pooled sensitivity and specificity for MRA were 73% and 88%, respectively, when using the clinical diagnosis as the reference standard [7]. Use of the MRA of cranial arteries is justified since it is now well‐documented that vasculitis is not limited to extracerebral arteries but may also extend to intracerebral arteries [6]. EULAR proposes MRA as the first imaging modality for the diagnosis of TKA. MRA assesses the vessel wall and luminal changes and distribution of the involved vessels. MRA sensitivity and specificity can be as high as 98% and 100%, respectively. Most commonly the left subclavian and common carotid arteries are involved. US can be used to examine the carotid, subclavian, and vertebral arteries, and abdominal aorta. Meta‐analysis of the role of US reported sensitivity and specificity of 81% and >90%, respectively, using clinical criteria and/or angiography as standard. In both TAK and GCA, MRA delineates the typical arterial mural abnormalities, provides an overview of involved arteries (both intracranially and extracranially), and is superior to US in examining the aorta [7]. Compared with MRA, FDG PET offers useful and complementary information about large vessel vasculitis. FDG PET/CT is considered a reliable imaging modality for the confirmation of LVV diagnosis. FDG PET detects inflammation in the affected arteries through detection of increased metabolism in the inflamed vessels. FDG PET/CT provides an overview of the disease extent in suspected cases. If the diagnosis is uncertain, PET/CT can evaluate alternative diagnosis such as tumors, lymphoma, or septic foci. On MRI, edema, thickening, and contrast enhancement of the vessel wall suggest active disease [6]; however, PET image findings seem to better correlate with the disease activity assessed clinically compared to the MRI findings. An additional advantage of PET is that both cranial and large vessels can be imaged in one session. On the other hand, luminal abnormalities such as stenosis, occlusion, and aneurysm can be evaluated by MRI, but are not well‐evaluated on noncontrast‐enhanced FDG PET/CT. Combining FDG PET and MRI in the form of hybrid PET/MRI provides simultaneous detailed morphological information of mural abnormalities together with metabolic activity. Arterial FDG quantification in LVV patients is comparable on PET/CT and PET/MRI, but disease extent on PET/MRI correlates better with the blood markers of systemic inflammation [5]. Figure 9.3 (A) Axial PET and fused PET/CT images for a 34‐year‐old male patient with history of large vessel vasculitis of the aorta externally compressing the coronary ostia showing diffusely increased mural uptake in the anterior aortic arch (blue arrows) and ascending aorta (green arrows). (B) Axial PET and fused PET/CT images showing increased mural uptake in the proximal left common carotid artery (blue arrows) and to a lesser extent the proximal innominate artery (green arrows). (C) Axial CT angiography images of the same patient demonstrating diffuse wall thickening of the aortic arch (blue arrow), ascending aorta (green arrow), proximal left common carotid artery (yellow arrow), and proximal left innominate artery (red arrow). At initial diagnosis, studies have shown that visual analysis alone is superior or equivalent to semiquantitative methods [7]. A scoring methodology – PETVAS score – has been proposed, and has been shown to correlate with the incidence of relapse. The score is derived from a visual assessment of uptake intensity using a scale comparing to liver (0 = no uptake, 1 = less than liver, 2 = equal to liver, and 3 = greater than the liver) in designated vascular segments (four segments of aorta, including ascending, arch, descending, thoracic and abdominal aorta, and in 11 branch arteries, including innominate, carotids, subclavians, axillaries, iliacs, and femorals). The final PETVAS score is then calculated as the sum of scores for each arterial segment [8]. The distribution of vessel involvement in GCA is the subclavian (73%), abdominal, and thoracic aorta (50%), with the axillary, carotid, iliac, and femoral arteries showing abnormal FDG uptake in 30–40% of cases (Figure 9.4). Specificity of FDG uptake is typically higher in supra‐aortic arteries and lower in the aorta and lower extremities as these arteries are more prone to atherosclerosis, which can also show uptake. The sensitivity and specificity of PET/CT in the initial diagnosis of GCA are reported to be as high as 92% and 85%, respectively, with temporal artery biopsy as a reference [6]. Figure 9.4 (A) Maximum intensity projection (MIP) of an FDG PET/CT study in a patient with GCA showing diffusely increased mural uptake in the bilateral subclavian arteries (purple arrows), axillary arteries (green arrows), and brachial arteries (blue arrows). (B) Axial PET and fused PET/CT images in the same patient showing increased mural uptake in the aortic arch (purple arrows), ascending aorta (blue arrows), and descending aorta (green arrows). (C) Additional coronal PET and fused PET/CT images also showing diffuse mural uptake in the bilateral common carotid arteries (purple arrows). (D) Coronal PET and fused PET/CT images in a patient with GCA showing diffuse mural uptake in the descending thoracic aorta (arrows). (E) Axial PET and fused PET/CT images in the same patient showing patchy mural uptake in the right axillary artery (arrows). (F) Coronal PET and fused PET/CT images of a follow‐up FDG study done 21 months later after receiving steroid therapy showing interval decrease in the intensity of uptake in the descending aorta wall indicating treatment response (arrows). (G) Axial PET and fused PET/CT images of the same follow‐up FDG study showing resolution of the uptake in the proximal right axillary artery (arrows). A significant number of patients with polymyalgia rheumatica (PMR) show increased large vessel vascular uptake suggestive of subclinical vasculitis. FDG PET imaging in PMR reveals perisynovitis with pathologic uptake in the soft tissues and ligaments around the joints and attachment sites of the shoulders and hips, lumbar and cervical spinous processes, and ischial tuberosities. In patients with GCA and PMR, increased uptake in the shoulders is seen and FDG uptake in the subclavian or axillary arteries is not more intense. When GCA is excluded, increased FDG uptake is seen in most patients and in the spinous processes of cervical/lumbar spine in half of the patients [7] (Figure 9.5). As PMR may be paraneoplastic, FDG PET can also identify the underlying malignancy [9]. FDG PET has a role in both the initial diagnosis and disease activity assessment in TAK [7]. Biopsies are not usually possible in most arteries involved in TAK, which heightens the need for accurate noninvasive diagnostic techniques [6]. In a metanalysis, FDG PET showed sensitivity and specificity of 87% and 74%, respectively, in differentiating active from inactive TAK [7]. TAK and GCA can have similar vascular distribution [10]. In TAK, the subclavian and carotid arteries and the aorta are most affected; involvement of abdominal aorta, and mesenteric and renal arteries is common [11]. In a recent metanalysis of FDG PET analyzing 21 studies with 413 patients in total, pooled sensitivity and specificity for GCA were 90% and 98%, respectively, and the sensitivity for TAK was lower at 84% [12]. The lower sensitivity for TAK may be because these patients tend to present in more chronic phase of disease when vascular wall inflammation has subsided. Figure 9.5 A 71‐year‐old woman was examined for nuchal pain, fatigue, and 10 kg weight loss in 5.5 months. She also complained of pain in the upper arms and shoulders, and she had noticed loss of strength in the shoulder and pelvic girdle, and jaw claudication. On clinical examination, blood pressure was equal in both arms, the left temporal artery was thickened with absent pulsations, the strength of the shoulder muscles was markedly diminished, and abduction of shoulders and hips was painful and difficult. Blood examination revealed a CRP level of 74 mg/L, ESR of 31 mm/h, hemoglobin level of 10.5 g/dL, and slightly increased alkaline phosphatase. Chest X‐ray and abdominal ultrasound results were normal. Clinical and biochemical features were suspicious for GCA and PMR. Vasculitis was confirmed by a positive temporal artery biopsy. FDG PET showed the typical features of both closely related conditions in this single patient: a strongly increased FDG uptake in the vessel walls of the carotid and subclavian arteries, and the aorta, revealing GCA, as well as an increased FDG uptake around both shoulders, hips, and between the spinous processes of the cervical and lumbar spine, revealing PMR. (Source: Reproduced from: Schmidt and Blockmans [7]) Intensity of FDG uptake in the thoracic aorta is an independent risk factor for complications of vasculitis such as aortic dilatation and aneurysm formation. In GCA, large vessel involvement is the strongest predictor of aortic dilatation. Patients with aortic complications of dilatation, aneurysm, and dissection nearly always have inflammation evident on the preceding FDG PET [7]. FDG PET/CT can be used for monitoring response to therapy in various vasculitides. FDG uptake decreases in response to successful treatment 4–12 weeks post therapy initiation [12]. Intensity of vascular uptake may decrease with glucocorticoid treatment; however, long‐term vascular uptake may still be seen despite clinical remission, which has been ascribed to vascular remodeling. Investigating the role of FDG PET for assessing disease activity in TAK is difficult considering the lack of gold standard in this disease condition [6]. FDG PET/MR is an attractive option for monitoring inflammatory processes in LVV. Because the two modalities detect different manifestations of inflammation, together PET/MR offers a comprehensive means of scoring the total burden of vasculitis. In TAK, combining PET and MR information has shown to determine disease stage based on mural and lumen morphology and inflammatory features [4]. PAN is a systemic necrotizing vasculitis involving medium and, to a lesser extent, small arteries. PAN affects multiple systems including the kidneys, nervous system, gastrointestinal tract, and skin. FDG PET can detect vasculitis in PAN and also nonvascular findings. For example, in cutaneous PAN FDG PET can sensitively detect disseminated foci throughout the subcutaneous and muscle tissue. FDG PET can detect chronic peri‐aortitis as an isolated finding or as part of IgG4 disease in which other organs such as pancreas, bile ducts, and lacrimal and salivary glands may also show abnormal uptake. In Behcet’s disease, pulmonary artery aneurysm and vasculitis of other large arteries can be seen on PET [7]. Figure 9.6 A 67‐year‐old woman with a granulomatosis with polyangiitis. FDG PET/CT scan shows increased FDG uptake in sinonasal and kidney locations (A and B, arrows). A follow‐up FDG PET/CT scan, although the patient achieved remission, showed resolution of hypermetabolic activities in both locations (C and D).
9
Vascular System
Introduction
Imaging of Vasculitis
Imaging of Large Vessel Vasculitis
Imaging of Medium and Small Vessel Vasculitis
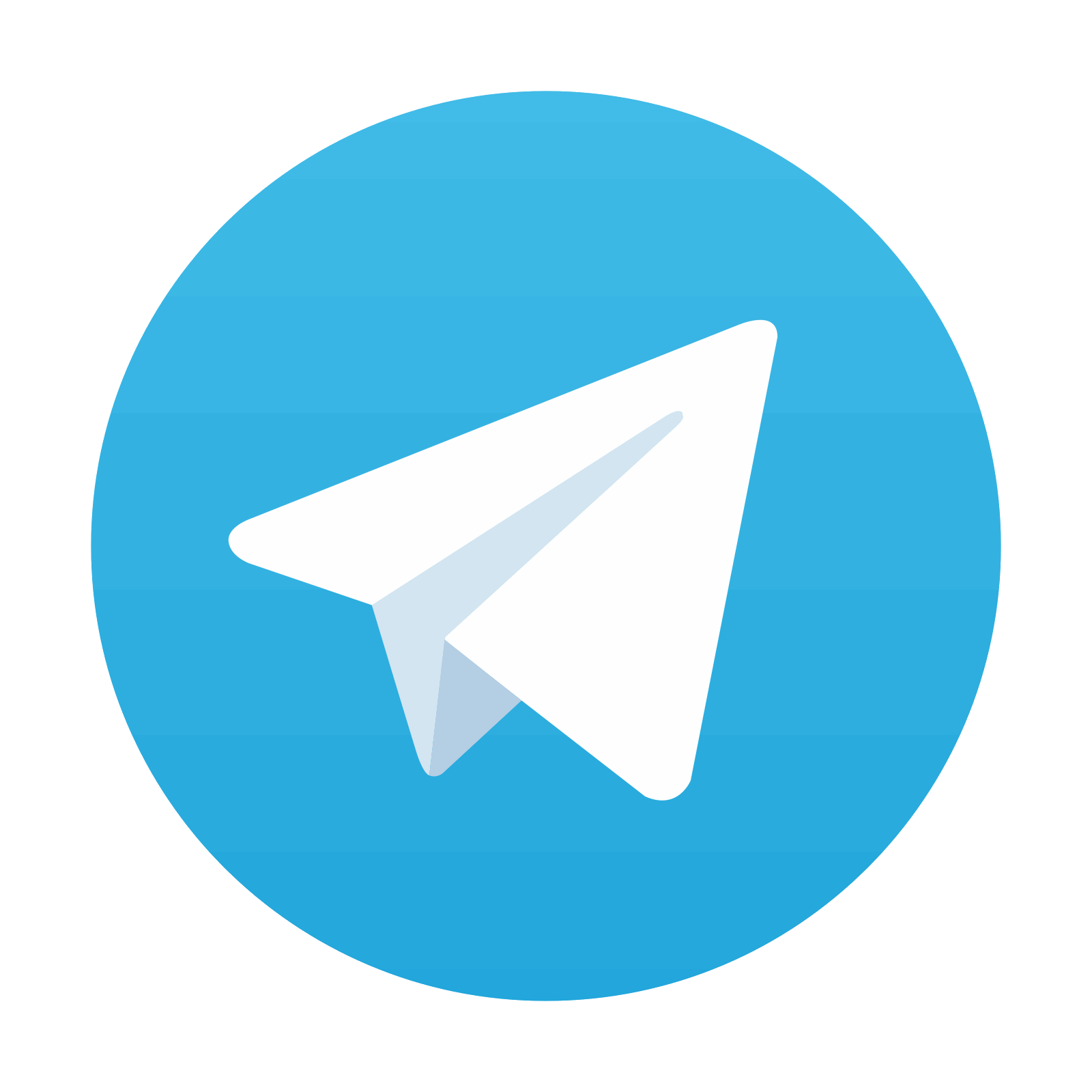
Stay updated, free articles. Join our Telegram channel

Full access? Get Clinical Tree
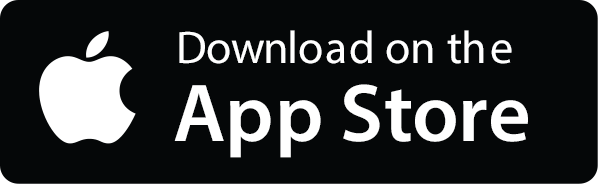
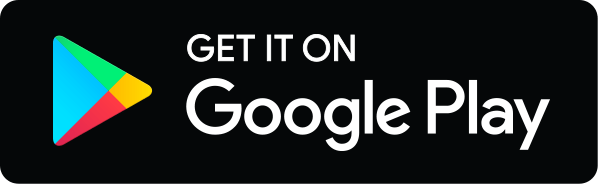