Fig. 15.1
Mesencephalic gait failure. MRI of an 83-year-old woman with gait failure after a hemorrhage in the locomotor mesencephalic region. Lesion is shown in the sagittal (a) and coronal (b) planes, arrow. (Reprinted with permission from Masdeu et al. [15])
Disequilibrium with “Automatic Pilot” Disorder
The disorders described next are characterized not only by disequilibrium, but also by a striking difference between the patient’s poor performance when they walk spontaneously and a better performance when they think about walking, for instance, by stepping over an obstacle or trying to take long strides. All of these lesions affect the corticobasal ganglionic–thalamo–cortical loop, described at the beginning of this section. The basal ganglia are part of an important loop that controls proximal movements participating in postural synergies.
1.
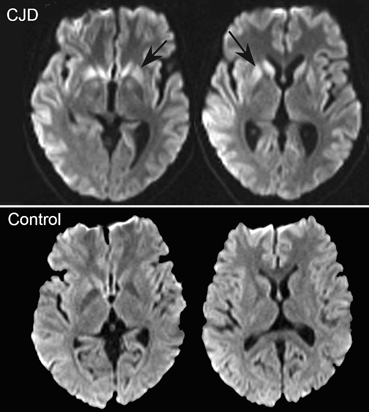
Basal ganglia lesions. Rarely, acute lesions of the basal ganglia may be associated with a syndrome of unsteadiness without the loss of isometric power, where a patient without an apparent weakness cannot, however, stand normally [16]. Disequilibrium and akinesia are also characteristic of advanced stages of disorders affecting the striatum, such as in the subcortical form of Creutzfeldt–Jakob disease (Fig. 15.2).
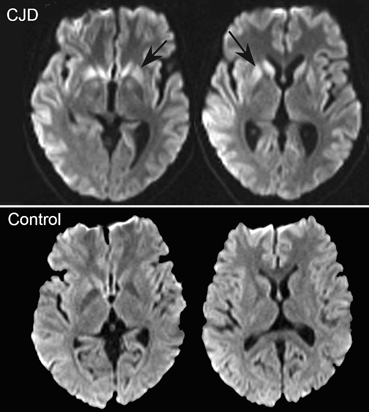
Fig. 15.2
Creutzfeldt–Jakob disease. Images shown (FLAIR MRI) are from a 39-year-old woman who developed chorea, followed by anxiety, progressive cognitive and motor impairment, leading to inability to walk and marked dysarthria and dementia by the time this imaging study was obtained, 5 months after symptom onset. Note the high intensity of the caudate and of the anterior portion of the lenticular nuclei (arrows). For comparison purposes, the lower image row was obtained with a similar technique, but from a control individual
2.
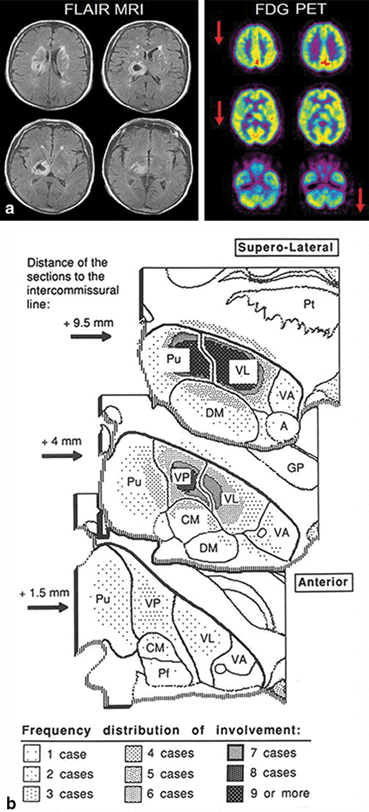
Thalamic lesions. Chronic lesions of the basal ganglia are better known to cause axial motor impairment than acute ones, whereas the opposite is true for thalamic lesions. A syndrome of impaired axial postural movements has been described with acute infarction or hemorrhage in the centromedian or ventrolateral nucleus of the thalamus or suprathalamic white matter (Fig. 15.3) [17–19]. Alert, with normal or near normal strength on isometric muscle testing and a variable degree of sensory loss or cerebellar ataxia, these patients cannot stand or even sit up unassisted for several days after an ischemic or hemorrhagic stroke. They fall backward or toward the side contralateral to the lesion. These patients seem to have a deficit of overlearned motor activity of an axial and postural nature. The syndrome has been called “thalamic astasia” and grouped by some among the central disequilibrium syndromes [6, 18].
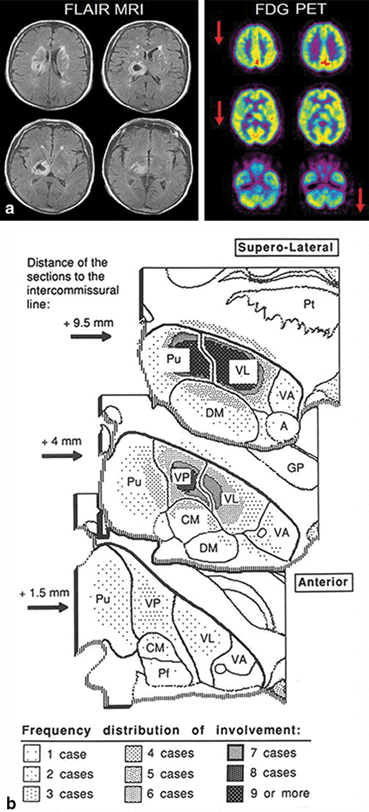
Fig. 15.3
Thalamic astasia. a Axial FLAIR MRI images showing a thalamic hematoma centered in the ventrolateral nucleus, from a 62-year-old man initially unable to walk, who at the time of this scan had marked gait unsteadiness, which improved when the patient concentrated on his gait. The 18F fluoro-deoxy-glucose (FDG) positron emission tomography (PET) study showed not only the deeply reduced metabolism at the site of the thalamic hematoma, but also reduced metabolism in ipsilateral frontoparietal cortex and contralateral cerebellum, including the vermian region. b Anatomic location of the lesion in 15 cases of thalamic astasia [18]. On successive axial sections of the thalamus, the frequency of involvement is indicated by the density of shading. Both right and left-sided lesions were plotted on the left thalamus. A anterior nuclear group, CM centromedian nucleus, DM dorsomedial nucleus, GP globus pallidus, Pf parafascicular nucleus, Pt putamen, Pu pulvinar, VA ventral anterior nucleus, VL ventrolateral nucleus, VP ventral posterior nucleus
3.
Hemispheric paracentral periventricular white matter lesions. The output of the thalamus critical for gait is directed to the areas of the cortex involved in lower extremity movements. This area of the cortex is the medial frontal region, specifically, the paracentral lobule and the supplementary motor cortex. The fibers reaching this area from the thalamus course through the periventricular white matter. Thus, it is not surprising that lesions here may result in impaired gait. Ischemic disease of the white matter is common in the elderly. Beginning with a report in 1989, many studies have confirmed that white matter abnormalities on computed tomography (CT) and MRI correlate with impaired gait unbalance in older people [20–22]. The kind of gait impairment seen most often in these patients corresponds to what has been termed the “cautious gait.” [23]. As they have poor balance, their steps are shorter, possibly to lessen the single-foot stance portion of the gait cycle. Like patients with thalamic lesions, these individuals may seem to walk rather normally so long as they pay attention to their gait. However, when they engage the “automatic pilot,” and the motor control system for involuntary movements begins to be relied upon, they tend to fall. Sudden buckling of the knees may precipitate them to the floor.
Disequilibrium may also be prominent in patients with hydrocephalus and with lesions in the medial aspect of the frontal lobe. However, those patients tend to have the gait disorder described further in this chapter as “magnetic gait.” Central disequilibrium is probably the commonest cause of the so-called “drop attacks,” sudden falls without warning or loss of consciousness in older individuals. Drop attacks were originally attributed to disease of the vertebrobasilar system, but this etiology of drop attacks in the elderly is probably not as common as subcortical hemispheric disease [24].
Freezing of Gait
With preserved balance, these patients experience gait hesitation and may freeze in the course of locomotion, particularly on a turn [5, 6]. Once the patient begins to walk, steps are short and shuffling, but become larger and the foot clearance increases as the patient continues to walk. The base of support is normal. Postural responses are preserved. Eye closure does not induce abnormal swaying. Maneuvers that bring about a “cortical strategy,” such as trying to kick an imaginary ball, step over a cane, or counting the steps help the patient initiate and maintain gait. Minus the disequilibrium, this disorder mimics the “automatic pilot disorder” described in the previous section. The anatomical localization of this disorder is still undefined, but involvement of frontobasal ganglionic mechanisms is suspected, because it shares features of the parkinsonian gait and the magnetic gait described next. It is likely that freezing is caused by lesions in a number of regions involved in gait [25]. The so-called “pure freezing of gait” is associated with a variety of progressive supranuclear palsy (PSP), a disorder where neuronal loss in the pedunculopontine nucleus has been documented [26–28]. Stimulation of the caudal pedunculopontine region improves freezing of gait in idiopathic Parkinson disease (iPD) , without necessarily improving or affecting other aspects of gait [14, 29, 30]. 18F-deoxy-glucose (FDG) PET has shown decreased midbrain metabolism in pure freezing of gait, whereas in PSP, there was additional involvement of the frontal cortex [31]. The involvement of the mesencephalic locomotor region has been supported by a study using blood-oxygen-level-dependent (BOLD) signal with functional MRI (fMRI) and volumetric measurements in patients with Parkinson disease without freezing and patients with freezing of gait [32]. Patients with freezing had atrophy and gait-related hyperactivity of the mesencephalic locomotor region; the hyperactivity correlated with the severity and duration of gait freezing (Fig. 15.4). However, changes were also detected in the medial frontoparietal region, in this [32] and other neuroimaging studies [33, 34], and a similar gait pattern has been described with lesions or atrophy in the medial frontoparietal region [35–37].
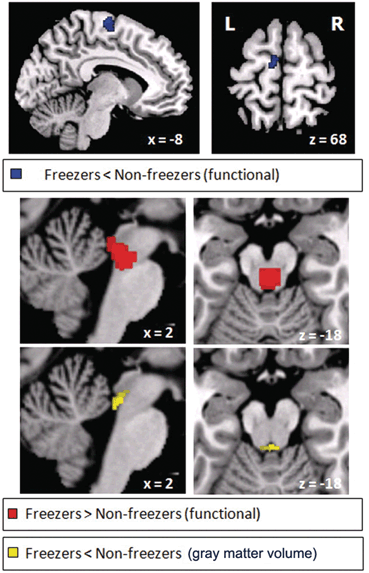
Fig. 15.4
Freezing of gait: brain correlates. Brain areas in which the relative increase in activity for motor imagery versus visual imagery differed between patients with freezing of gait (freezers) and patients without freezing of gait (nonfreezers). Freezers had decreased activity in the supplementary motor cortex (region of interest analysis, P = 0.06 corrected for multiple comparisons using family-wise inference on voxel level) and increased activity in the mesencephalic locomotor region (whole brain search, P < 0.05 similarly corrected for multiple comparisons); the corresponding statistical parametric maps are shown superimposed on standard brain sections (top: supplementary motor cortex, middle: mesencephalic locomotor region). The bottom brain sections show in yellow the small cluster with significantly smaller gray matter volume in patients with freezing of gait in the mesencephalic locomotor region. (From Snijders et al. [32])
Magnetic Gait
This disorder corresponds to what Nutt et al. [6] call “frontal gait disorder” and others have described as “marche à petit pas” or “arteriosclerotic parkinsonism.” Meyer and Barron called it “apraxia of gait,” because despite the severe gait disorder the patients can move their legs at will, lacking any sensory loss or weakness on isometric strength testing [38]. Although able to stand, these patients have such an inability to lift their feet and walk that their feet may seem to be glued to the floor. Some patients have great difficulty initiating walking and, when pushed forward, the heels are lifted but the toes seem to grab the floor. There may be a dissociation between gait and distal volitional movements, in that the patient may be quite able to draw figures with her feet, or to do normally the heel-shin maneuver. For this reason, some authors argue that calling this disorder “apraxia” is inappropriate, because in apraxia complex motor sequences, such as to draw a figure, are maximally affected [39, 40]. Milder forms of the same disorder resemble the parkinsonian gait, with short, shuffling steps and truncal rigidity. Arm swing during walking may be preserved or even exaggerated and if so, helps differentiate this disorder from iPD [37]. The turns are very slow and broken down into many steps. Turning may bring up the tendency for the feet (or for one foot more than the other when the problem is asymmetrical) to become glued to the floor. Freezing may become evident, as the steps halt and the patient remains motionless or develops tremor-like movements of the lower legs. Falls are common, particularly in patients who have disequilibrium. This disorder may be caused by bilateral lesions of the medial frontal cortex (Fig. 15.5) [36, 37], severe hydrocephalus, or bilateral ischemic lesions of the white matter. Hydrocephalus and ischemic lesions will be discussed in the next section on Etiology.
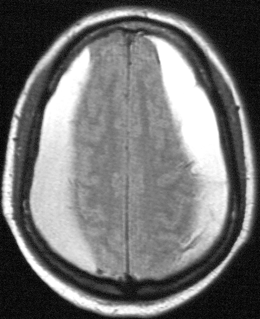
Fig. 15.5
Subdural hematomas. Axial FLAIR MRI image showing bilateral convexity subdural hematomas in an 82-year-old man with gait freezing and an attentional disorder
Etiology of Complex Gait Disorders
Having discussed the phenomenological classification and some of the neuroimaging findings in gait disorder, the next section includes a description of the neuroimaging findings in the three most common causes of gait disorders in adults, namely, cervical spondylosis, vascular brain disease, and hydrocephalus.
Cervical Myelopathy from Cervical Spondylosis
Cervical myelopathy from cervical spondylosis has been reported as the second most common cause of gait impairment in the older adult [41, 42]. As it is potentially treatable, cervical myelopathy should be considered in any older adult presenting with gait impairment [43]. Some patients present with the characteristic clinical picture of cervical radicular pain and spastic paraparesis , but most do not have radicular pain. Initially, at the time when surgery is most likely to be successful and prevent further deterioration, the gait impairment may be very subtle, noticeable to the patient but yielding minimal findings on neurological examination [44]. The paucity of findings may delay the diagnosis. In the Yale series, the investigators felt that the diagnosis had been delayed in the average by 6.3 years [44]. The earliest consistent symptom in all of their 22 patients was a gait abnormality. Early diagnosis is important, because the myelopathy of cervical spondylosis is often progressive if untreated [44].
MRI of the cervical spine is helpful in the diagnosis of cervical myelopathy from cervical spondylosis , but, in the absence of the clinical findings, the presence of spondylitic changes by themselves does not make the diagnosis. Rather severe changes can be seen in asymptomatic older adults [45–47]. Baseline plain x-rays are useful when surgery is being considered for comparison with postoperative x-rays or when the patient cannot undergo MRI, for instance, in the case of someone bearing a pacemaker. However, MRI is the screening test of choice in most instances [44]. Boney osteophytes can be seen as black ridges compressing the canal and, if the compression is severe, causing high-intensity changes in the spinal cord on T2-weighted images (Fig. 15.6).
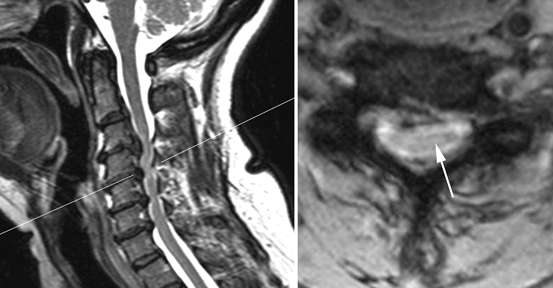
Fig. 15.6
Cervical myelopathy from spondylosis. Sagittal (left) and axial (right) MRI images from a 61-year-old man with an ataxic-spastic gait, showing spinal cord compression by osteophytes at the C3-C6 levels. At those levels, the spinal cord is abnormally hyperintense in these T2-weighted images (detail on the right image, arrow). The level of the axial image, at the C4-C5 disc level, is indicated by a thin line on the sagittal image
In addition to the narrowing of the spinal canal by spondylitic hypertrophy of bones and ligaments, two changes are frequently observed in the cord itself: decreased diameter and a hyperintense signal on T2-weighted images. How often a hyperintense area is observed depends on the stage of progression, with gliosis and microcavitation of the gray matter, and other poorly understood factors. In some series, as many as 40 % of the patients have this finding [48]. Some have found the presence of this finding and its irreversibility after surgery to predict a poor outcome, while others have not [48, 49]. MRI may show other changes within the spinal cord itself due to chronic compression such as syrinx formation. MRI is also of great value in the differential diagnosis of myelopathy, because conditions such as tumor and infection can be easily ruled out.
Although MRI is not as good as CT in showing osteophytes or ossification of the posterior longitudinal ligament [50, 51], it has replaced CT almost completely in the evaluation of cervical spondylosis [52]. Very rarely, thin-slice CT of the cervical spine after intrathecal introduction of a nonionic water soluble contrast material may be of help (Fig. 15.7).
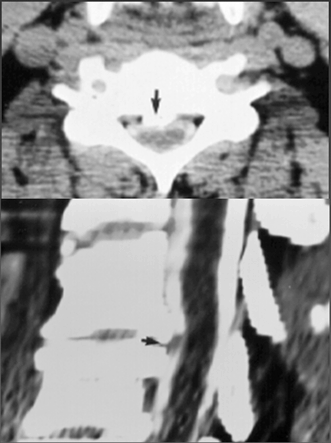
Fig. 15.7
Posterior longitudinal ligament calcification. Axial (top) and sagittal (bottom) CT images of the cervical spine, obtained after the infusion of metrizamide in the subarachnoid space through a lumbar puncture. Note that the bony details are better visualized with CT than with MRI (see Fig. 15.6). But changes in the spinal cord itself are better seen with MRI (see Fig. 15.6)
Vascular Brain Disease
Vascular disease of the brain ranks among the three top causes of gait impairment in the older adult [41, 42]. These are patients referred for progressive gait impairment; here we are not discussing patients with a stroke that leaves them with hemiparesis and gait difficulty. Ischemic brain disease tends to follow one of three patterns, discernible by clinical evaluation and with the help of neuroimaging procedures: (1) cortical infarcts, most often related to embolic disease; (2) subcortical disease, often in the form of widespread lacunes and white matter changes, most often related to arteriolar disease; and (3) a mixture of the two patterns, often related to atheromatous disease of the major vessels [24]. Subcortical infarcts strategically located may impair equilibrium and gait with little or no limb weakness on isometric testing [24]. Although acute stroke tends to be associated with overt neurological findings, gait impairment may be neglected as a neurological finding and yet be the result of small, “silent” lacunar strokes [53]. Vascular disease causing this syndrome most often affects the supratentorial compartment in the form of lacunar disease or ischemic disease of the white matter. Less often, it affects the posterior circulation, involving vestibular or cerebellar structures, and very rarely, other brainstem structures important for gait, such as the mesencephalic locomotor center (see Fig. 15.1).
1.
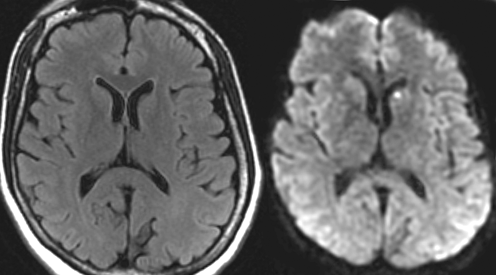
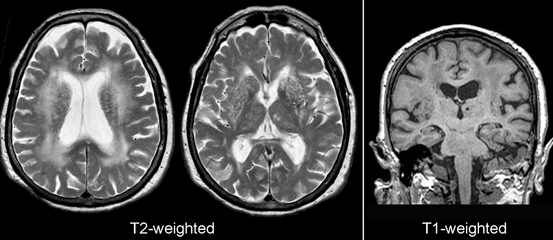
Lacunar disease of gait-critical structures. CT and MRI are the diagnostic tests most useful for the study of these syndromes. Hemorrhagic lesions can be readily detected by either modality, whereas acute lacunar lesions may pass unnoticed unless MR diffusion imaging is used (Fig. 15.8). Very small older lacunes may also be missed, because the infarcted tissue retracts and healthy tissue takes up its place. The resulting atrophy can be quantified using region of interest (ROI) methods or voxel-based morphometry [54, 55]. Larger lacunes can be easily appreciated on MRI as elongated lesions, following the trajectory of the arteriolar perforators, bright on T2-weighted images, including fluid-attenuated inversion recovery (FLAIR) images, and dark on T1-weighted images (Fig. 15.9). Although in the supratentorial compartment small lacunes are easier to spot on FLAIR images, conventional T2-weighted images are superior for lesions in the brainstem [56]. Supratentorial lacunes most likely to cause gait and balance problems affect the ventrolateral nucleus of the thalamus, suprathalamic white matter, basal ganglia, and the paracentral white matter. In order to cause persistent impairment, the lesions have to be bilateral and reach a volume threshold. The correlation of the imaging data with the clinical findings is often difficult because the gait impairment caused by these lesions affects the unconscious control of gait and posture (the “automatic pilot”) [18, 57]. Often, patients with a shuffling, unsteady gait at home perform normally at a physician’s office: they are successfully engaging the cortical mechanisms of gait to compensate for the impaired subcortical mechanisms. Here, the history, coupled with the neuroimaging findings, can be most helpful [58].
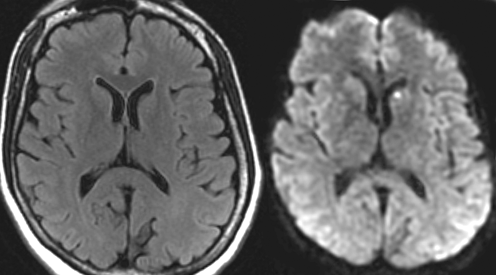
Fig. 15.8
Acute lacunar stroke. MRI from a 60-year-old man with a left caudate nucleus infarct, visible on the diffusion-weighted image (right), but not on the acute FLAIR image (left)
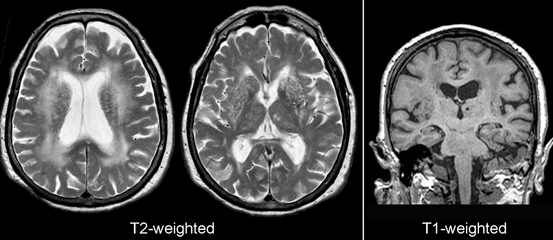
Fig. 15.9
Microvascular white matter disease. Shown are axial T2-weighted and coronal T1-weighted images from an 80-year-old woman with impairment of cognition and gait. Note the thalamic infarctions and the large areas of altered signal (increased on T2 and decreased on T1) in the centrum semiovale. The thalamic lacunes are bright on T2 and dark on T1-weighted images
2.
White matter disease . White matter changes on CT or MRI are very frequent in older people (see Fig. 15.9). On MRI, some degree of white matter change is present in about 95 % of subjects over the age of 60 and increases with age [59, 60]. Areas in the hemispheric white matter are hypodense on CT and markedly hyperintense on T2-weighted images and FLAIR, and hypointense on T1-weighted images (see Fig. 15.9). However, high-intensity areas on T2-weighted MRI are often undetected on CT. On T2-weighted MR images, it may be difficult to differentiate tissue damage from increased water content due to dilation of perivascular spaces with normal aging because both have increased intensity values [61]. For this reason, in older persons it seems preferable to quantify lesions visible on T1-weighted images or CT as indicators of true white matter damage. However, there are little data available in this age group exploring the functional significance of white matter changes visible only on T2-weighted images versus those visible on both T2 and T1-weighted images. In multiple sclerosis, and therefore in a younger age group, it is known that T1 lesion volume correlates better than T2 volume with hemispheric dysfunction [62]. T1-mapping, which determines tissue-specific T1-relaxation times, has been used recently to correlate postmortem changes [63, 64]. It may reflect pathological processes related to intraparenchymal changes in water content such as edema, widening of the extracellular space, subtle blood–brain barrier leakage, or glial proliferation [63, 64].
Quantification of White Matter Disease
A number of techniques are available to evaluate the extent of white matter involvement based on intensity changes. Initial methods were semiquantitative, and involved the visual inspection of images. Changes could be compared among individuals, on a cross-sectional basis, or longitudinally in the same individual. Cross-sectional comparisons have been made by simply grouping the scans of patients and controls from 1 (least amount of white matter changes) to 8 (greatest amount) [22]. Most often used are scales describing the amount of white matter changes. The Fazekas Scale [65] has a range of 1–6. In the periventricular regions scores 0–3 can be given, and in the subcortical region scores 1–3 can be given for mild, moderate, or severe lesions, respectively. The age-related white matter changes (ARWMC) scale [66] has a range from 0 to 30, where scores 0–3 can be given in 5 regions, left and right each. The Scheltens Rating Scale [67] ranges from 0 to 84 (scores 0–6 can be given in 13 subcortical regions and scores 0–2 for three periventricular regions). An example of longitudinal scale is the Rotterdam Progression Scale [68] (range − 7 to 7), which measures decrease, no change, or increase (− 1, 0, 1, respectively) of white matter changes for three periventricular regions and four subcortical regions. These visual methods are giving way to semiautomatic [69] or even completely automatic methods, which use the matrix output from the scanner to select voxels likely to correspond to abnormal white matter [70]. Ideally, images to be processed automatically should be obtained with good magnetic field homogeneity or with an additional sequence for inhomogeneity correction, small voxel size (1–2 mm3), and with 3D acquisitions, where the entire brain is imaged with voxels having the same size in all three planes. In good quality scans, automatic or semiautomatic segmentation methods can measure the extent of white matter changes accurately and with a minimum of operator effort [71]. Not only does the global amount of white matter changes, but their location can also be assessed automatically [72].
Diffusion tensor imaging (DTI) has been extensively applied to the study of white matter changes in older people [73–78]. DTI measurements indicating white matter damage, particularly decreased functional anisotropy and increased radial diffusivity, have been found at autopsy to correlate with white matter ischemic lesions, free-radical injury, and aberrant oligodendrocytes [79]. Not surprisingly, there is a better correlation between DTI measurements and intensity on FLAIR in those areas likely to be mildly to moderately affected, such as those located farther from the ventricles [80]. The correlation is lost in the periventricular region, with very high FLAIR values. DTI measurements of the corpus callosum have been used in the study of gait [81]. The corpus callosum affords an easy and appropriate target for DTI studies, because most fibers run parallel to each other and there is very little fiber crossing. In regions where major pathways cross perpendicularly, such as the superior longitudinal fasciculus and the corticospinal tract, axonal loss in one of the pathways may actually result in an increased anisotropy, as more of the remaining fibers are parallel to each other [82]. As regards gait, genu DTI abnormalities may simply reflect the involvement of frontal pathways by the disease process and the callosal fibers may have little or nothing to do with gait. DTI findings in participants with a range of microvascular brain disease must be interpreted with caution, because hemodynamic changes, regardless of the integrity of white matter, can affect fractional anisotropy and other DTI parameters [83].
As the histology underlying white matter changes in older people remains elusive in many cases, Hachinski [84] coined the descriptive term “leuko-araiosis” for the CT findings and this term was later on applied to the MRI findings as well. Many authors use other purely descriptive terms, such as white matter hyperintensities [58]. Several authors have reported normal histology, but ischemic changes similar to the findings in subcortical arteriosclerotic encephalopathy (SAE), have been present in cases with pronounced changes on T1-weighted MRI or CT [85, 86], or even T2 changes [87]. Microglial proliferation, arteriolar thickening, and biochemical indicators of tissue hypoxia are present within regions with abnormal MRI signal [87, 88]. Attempts at remyelination have been detected in periventricular but not subcortical lesions [88]. Cerebrovascular disease and hypertension are frequent correlates of SAE [89, 90]. Amyloid angiopathy can also cause white matter disease [91, 92]. In addition to white matter changes, amyloid angiopathy results in subcortical hemorrhages (Fig. 15.10).
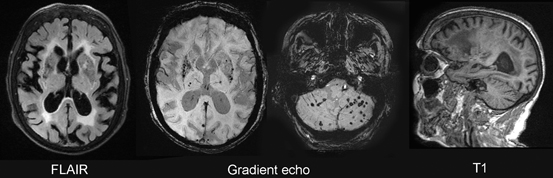
Fig.15.10
Cerebral amyloid angiopathy (CAA). Shown are MR images from a 72-year-old woman with dementia and CAA. The white matter contains many abnormal areas, which appear hyperintense on the transverse FLAIR image and hypointense on the sagittal T1-weighted image. Multiple lacunar infarcts are present in the lenticular nuclei and few in the thalami. Microbleeds, best seen on the gradient echo images, dot the lenticular nuclei, thalami, and the cerebellum. Scattered microbleeds can also be seen in the cortex or subcortical white matter
Disorders of gait and equilibrium are the most prominent correlate of white matter CT or MRI changes in older people [24, 53, 57]. In controlled studies of elderly prone to falling, impaired gait and balance correlated with the presence of white matter disease on CT or MRI [22, 58]. Studies relating gait to DTI changes are scarce. In a study of 173 community-dwelling elderly, DTI measurements in the genu of the corpus callosum correlated with gait function measured by the Tinetti gait and balance scores [81].
Hydrocephalus
This section will refer to chronic hydrocephalus in the adult, and not to pediatric hydrocephalus, or to hydrocephalus secondary to head trauma or aneurysmal subarachnoid hemorrhage. Although at an annual incidence of 1.8 cases/100,000 inhabitants [93], symptomatic hydrocephalus is not a frequent cause of gait disorder, it presents initially with a gait disorder and should be recognized because it is potentially treatable [94, 95]. Patients with hydrocephalus have a variety of gait disorders, but slowing of gait, short steps, and balance disturbances have been recorded most often [96–98]. Start hesitation and freezing of gait are occasionally observed [99]. In severe cases, a magnetic gait may be present. In some series, instability was associated with a poor response after shunting [100]. Balance has been recorded to improve after shunting or cerebrospinal fluid (CSF) drainage in some series [99, 101], but not in others [98]. It has been postulated that the best predictor of shunting success is the response to CSF withdrawal: if gait is observed to improve after CSF removal, a good response is likely [95]. However, as gait improves when cortical strategies, rather than the “automatic pilot” are used, patients often do walk better after CSF has been removed and they are “expected” to walk better. This placebo effect has been documented in individual cases [102]. For this reason, more objective structural neuroimaging findings are very helpful.
Enlarged ventricles are frequently present in patients with gait disorders [103] and are associated with gait impairment [104]. From these data, some authors concluded that symptomatic hydrocephalus was common and shunting procedures multiplied in the last three decades. However, even in series with carefully selected patients, some failed to improve after shunting or only had a short-lived improvement, suggesting that hydrocephalus was not the cause of their gait disorder [100, 105]. For this reason, it is important to apply more sensitive diagnostic criteria in the work up of these patients [94]. In the opposite end of the spectrum, patients with gait or balance impairment caused by symptomatic hydrocephalus may not be shunted because the MRI shows large sulci, giving the impression of hydrocephalus ex vacuo (Fig. 15.11).
< div class='tao-gold-member'>
Only gold members can continue reading. Log In or Register a > to continue
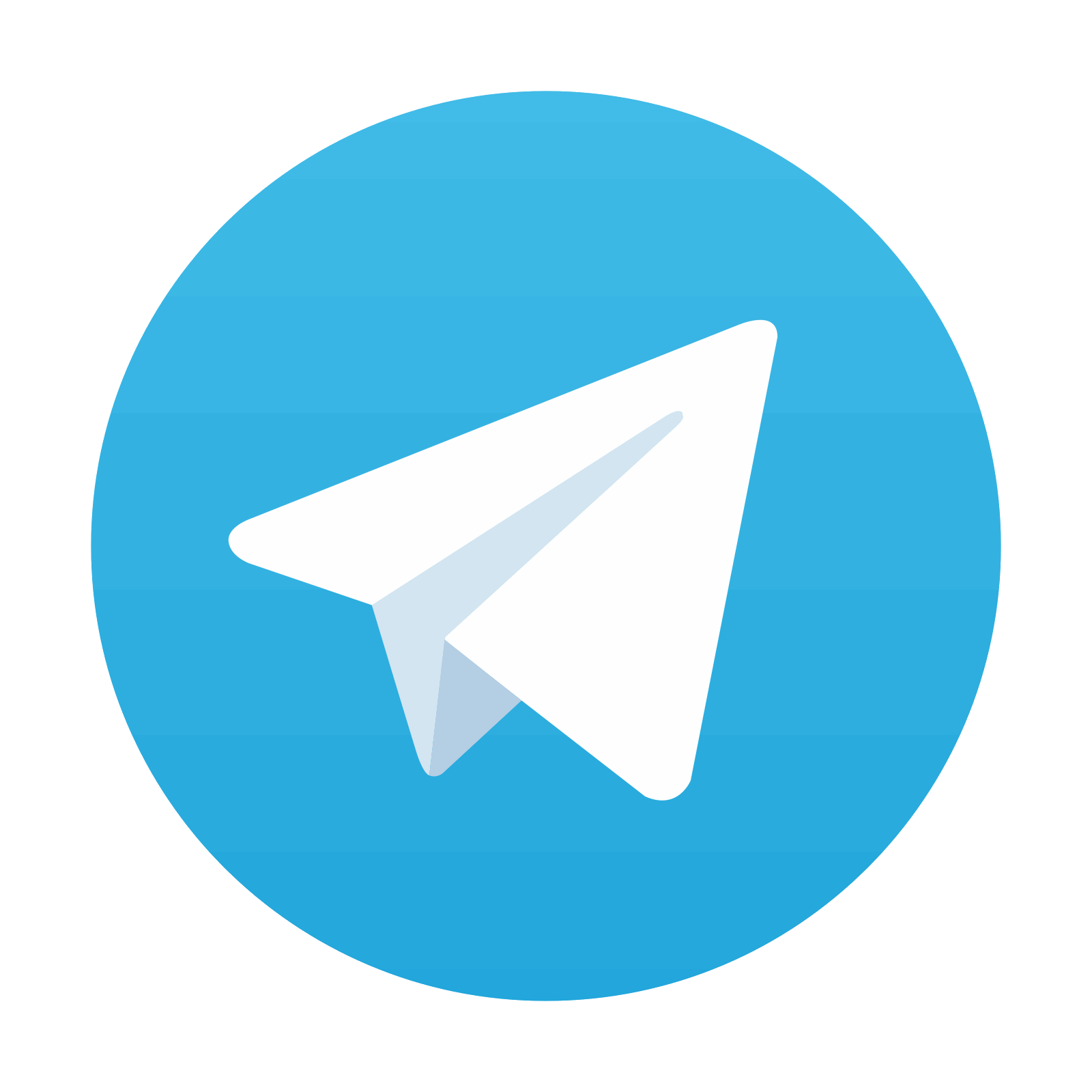
Stay updated, free articles. Join our Telegram channel

Full access? Get Clinical Tree
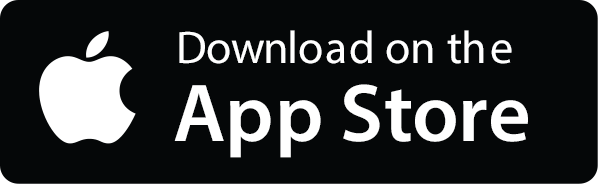
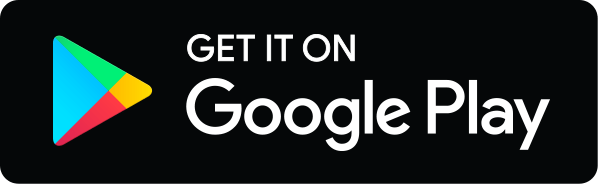